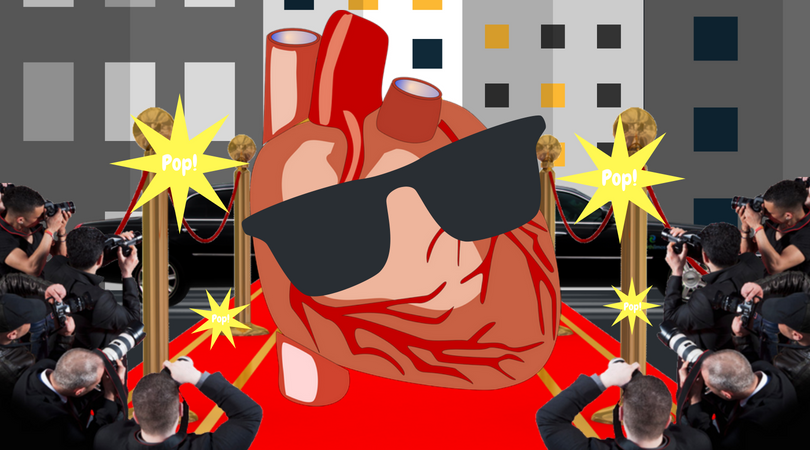
Basics of Heart Anatomy & EKG
EKG can be a difficult concept to understand. Many healthcare providers struggle with EKG because of the inadequate professors and/or lectures they had during their college years. In actuality, EKG is very easy. In fact, the secret to understanding EKG is to have a GREAT instructor or professor. However, to better understand EKG’s, healthcare providers must first possess a better understanding of heart anatomy and certain key terms. In all honesty, most EKG instructors expect students to already know heart anatomy and cardiac terms, and accordingly, many instructors will choose to omit these concepts. To facilitate the learning of EKG, this article will provide more insight into essential key terms to better understand how the heart conducts then contracts.
Stuff you Already Know
The Cardiovascular System consists of the heart, blood, and blood vessels. The heart is the hardest working muscle in the body as it beats non-stop 60 to 100 beats per minute pumping blood throughout the blood vessels by repeated, rhythmic contractions. In addition, the heart is located in the mediastinum, which is in the middle of the thoracic cavity and behind the sternum. In the majority of the population, the apex points towards the left.
Moreover, the pericardium encloses the heart for protection. The pericardium contains serous fluid which serves as a lubricant to reduce friction from muscle movement. A network of blood vessels carries oxygen and nutrients to all the tissues of the body. Arteries are the blood vessels that take blood away from the heart. Whereas, veins are the blood vessels that return blood to the heart. Capillaries are very small blood vessels that lie between the arteries and veins.
This seems like too much information to just throw in a paragraph. To facilitate all this information, let’s break down the heart’s anatomy into smaller parts.
Layers of the Heart
The heart is made-up of three layers, the epicardium, myocardium, and endocardium.
Epicardium
- The epicardium, also called the pericardium, is the outermost layer of the heart.
- This 2-layer sac contains the fibrous and serous pericardium.
- The fibrous pericardium keeps the heart in place and prevents overfilling.
- The Serous pericardium contains two layers, the parietal layer and visceral layer.
- Between both layers, there is a serous fluid that helps to prevent friction.
- This 2-layer sac contains the fibrous and serous pericardium.
Myocardium
- The Myocardium is the thick, muscular middle layer that contains cardiac muscle cells, which are responsible for contraction. On a side note, cardiac muscle cells DO NOT make an impulse of electricity, nor do they carry electricity, in contrast, they are only responsible for cardiac muscle contraction.
- A specific property of cardiac muscle cells is contractility. Contractility is the strength or force of contraction.
- Contractility has to do with the cardiac muscle cells ability to shorten or lengthen their myosin filaments, or muscle fibers.
- To put this another way, contractility is the ability of cardiac muscle cells to contract in the ventricles after depolarization.
- Contractility depends on the muscle fibers ability to stretch. As the blood enters the ventricles at the end of diastole, the blood entering causes the muscle fibers to stretch.
- For example, think of a rubber band. Picture trying to shoot a rubber band across the room, if you don’t stretch it enough, it won’t go far. If you stretch it too much, it will snap, however, if you stretch it just the right amount, it will go as far as you want it to.
- This is how contractility works.
- Another specific property of cardiac cells is extensibility. Extensibility is the cardiac muscle cells ability to stretch.
- A specific property of cardiac muscle cells is contractility. Contractility is the strength or force of contraction.
Endocardium
- The innermost layer is the Endocardium.
- This layer lines the chambers and provides protection to the valves and heart chambers.
Chambers of the Heart
The heart is made of 4 chambers, the right and left atrium, and the right and left ventricles.
Atria
- The right atria receives deoxygenated blood from the inferior and superior vena cava.
- On the other hand, the left atria receives oxygen rich blood from the pulmonary veins.
Ventricles
- The right ventricle pumps deoxygenated blood to the pulmonary artery which then travels to the lungs to pick up O2 and ultimately drop off CO2.
- The left ventricle pumps oxygenated blood toward the aorta which then travels to all body tissues.
- Afterload is the amount of pressure the left ventricle must work against to pump blood into circulation. The greater the resistance, the more the heart works to pump out blood. To illustrate, think about a balloon analogy, afterload is represented by the knot at the end of the balloon. To get the air out, the balloon must work against that knot.
Heart Valves
The heart contains 4 valves that prevent the backward flow of blood into the chambers.
Atrioventricular valves
- Valves that separate the atria from the ventricles are called the atrioventricular valves.
- The tricuspid valve on the right side of the heart prevents blood entering the right ventricle from flowing back into the right atria.
- The mitral valve on the left side of the heart prevents blood entering the left ventricle from flowing back into the left atrium
Semilunar valves
- Valves at the ventricular outlets are called semilunar valves.
- The pulmonary valve prevents blood entering the pulmonary artery from flowing back into the right ventricle.
- Alternatively, the aortic valve prevents blood entering the aorta from flowing back into the left ventricle.
Cardiac Cycle
A single cycle of cardiac activity can be separated into two phases, systole and diastole.
Systole
- Systole means contraction. In simple terms, systole is the phase of the heartbeat where the heart muscle contracts and pumps blood to the chambers or out to the body.
Diastole
- In contrast, Diastole means relaxation. In other words, diastole is the phase of the heartbeat where the cardiac muscle relaxes and allows the chambers to refill with blood.
- Preload signifies how much the ventricles stretch at the end of diastole.
- To illustrate, when blood returns to the heart via the venous system, and enters the ventricles, the sarcomeres that make up the ventricles will stretch. If venous return is high, as in a lot more blood coming in, the sarcomeres will stretch more and there will be more ventricular refilling, ultimately increasing the preload.
- However, if blood return is low, as in hypovolemia, the sarcomeres will not stretch enough which will lead to less ventricular refilling, ultimately decreasing the preload.
- Preload signifies how much the ventricles stretch at the end of diastole.
Conduction System
Systole and Diastole occur because of the heart’s electrical system, also called the conduction system. In particular, the heart’s conduction system transmits cardiac impulses which cause the heart to beat. This is NOT nervous tissue.
Electrical cells
- The electrical system is made up of specialized cells called electrical cells.
- The electrical cells make up the nodes and conducting fibers of the conduction system. Electrical cells possess the following properties:
- Automaticity which is the electrical cells ability to spontaneously generate and discharge an electrical impulse.
- Excitability which is the ability of the cell to respond to an electrical impulse.
- Conductivity which is the ability to transmit an electrical impulse from one cell to the next.
- The electrical cells make up the nodes and conducting fibers of the conduction system. Electrical cells possess the following properties:
Electrical Activity
- This electrical activity can be categorized into two categories, depolarization and repolarization.
- Depolarization is the electricity as it moves down the conduction system.
- For example, when the sinus node makes an impulse of electricity, this electricity will begin to travel down toward the AV node, the electricity as it moves from the sinus node to the AV node is called depolarization. At the same time the cardiac muscle cells will begin to contract. We say that depolarization causes systole, which is contraction.
- Repolarization, on the other hand, is when there is no electricity because the ions that created the electrical impulse in the first place returned to their resting state.
- To simplify, depolarization cannot be continuous. When the electricity moves down the conduction system what is left behind is a state of no more electricity. This is repolarization, no electricity means that the heart muscle is in diastole, or in a resting state.
- Depolarization is the electricity as it moves down the conduction system.
The Sinus Rhythm
A sinus rhythm produces a P wave, a Complex, and a T wave. In a healthy individual, the sinus rhythm indicates atrial contraction, as represented by a P wave; as well as, ventricular contraction, as represented by a QRS complex; and ventricular resting, as represented by a T wave.
Sinus Node
- The SA Node (“Sinus Node”) is the heart’s dominant pacemaker, and its pacing activity is known as a “Sinus Rhythm.” The SA Node initiates a depolarization wave at regular intervals. This wave proceeds outward and stimulates both atria to contract.
- For instance, imagine the atria as a pool of water. A pebble dropped in at the SA Node produces an enlarging, circular wave that spreads outward.
- Atrial depolarization is a spreading wave of positive charges within the atrial myocardial cells.
- Each depolarization wave emitted by the SA Node spreads through both atria, producing a P wave on the ECG.
- So when we see a P wave on an electrocardiogram, we know that, electrically speaking, it represents atrial contraction.
- This atrial depolarization moves through both atria and therefore, both atria contract simultaneously.
AV Node
- The AV node’s main function is to delay impulses by 0.04 seconds to keep the ventricles from contracting too quickly.
- This delay allows the ventricles to complete their filling phase as the atria contract.
- It also allows the cardiac muscle to stretch to its fullest for peak cardiac output.
- The impulse delay that occurs in the AV node is referred to as the P-R interval.
Bundle of HIS
- The rapid conduction impulse now moves into the ventricles by way of the bundle of HIS, then the bundle branches, and finally the Purkinje fibers.
- The bundle of HIS first carries the electricity into the intraventricular septum.
- This bundle of HIS divides into the right and left bundle branches, therefore causing the electricity to bifurcate into the right and left bundle branches.
- The movement of electricity down the bundle on HIS begins to produce the QRS complex.
- This bundle of HIS divides into the right and left bundle branches, therefore causing the electricity to bifurcate into the right and left bundle branches.
- The bundle of HIS first carries the electricity into the intraventricular septum.
Bundle Branches
- The right bundle branch carries the electricity down the right side of the intraventricular septum and then through the right ventricle.
- The left bundle branch, on the other hand, carries the electricity down the left side of the intraventricular septum and through the left ventricle.
- The left bundle branch splits into two branches that extends through the lateral and the posterior portions of the left ventricle.
- The movement of electricity down the bundle branches further develops the QRS complex.
- The left bundle branch splits into two branches that extends through the lateral and the posterior portions of the left ventricle.
Purkinje Fibers
- Lastly, the Purkinje fibers carry the electricity from the bundle branches into the endocardium, then deep into the myocardial tissue.
- Depolarization of the entire ventricular myocardium produces a QRS complex on the ECG.
Finally, the QRS complex actually represents the beginning of ventricular contraction also referred to as ventricular depolarization.
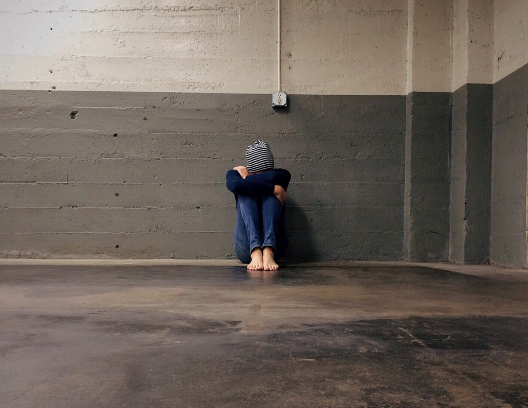